CLICK THE PLAY BUTTON TO WATCH THE LESSON.
Action list:
In this lesson, we will take a closer look at how the return current in a circuit behaves as the frequency changes and how these changes affect the layout of a printed circuit board (PCB). We will also examine how these variations can influence key aspects such as electromagnetic compatibility (EMC) and signal integrity.
Understanding how frequency impacts the return current is essential for designing PCBs that perform well in different conditions and meet necessary standards. By gaining insight into these factors, we can make better decisions in the design process, ensuring that our boards work efficiently and reliably.
Let’s start by looking at a simulation created with Simbeor ®, a specialized software for electromagnetic and signal integrity simulations. In this simulation, we see a signal trace on the top layer of the PCB and a return reference plane positioned directly underneath it.
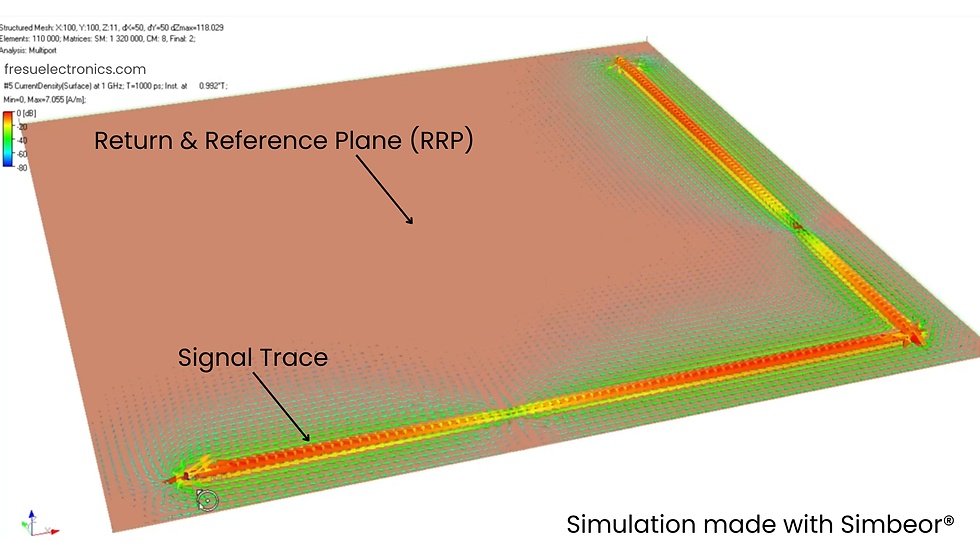
This simulation essentially replicates the conditions encountered when designing a PCB, as shown in this example in Figure 2. In this case, we have the top layer, where the signal traces are placed (shown in red), and beneath it, a return plane (shown in green).
This return plane is continuous and uninterrupted, without any cuts, splits, or breaks. It provides a solid reference for the signals on the top layer, ensuring that the return path for the signals remains consistent and well-defined throughout the PCB.